Brain wrinkles and folds matter – researchers are studying the mechanics of how they form
- Written by Mir Jalil Razavi, Assistant Professor of Mechanical Engineering, Binghamton University, State University of New York
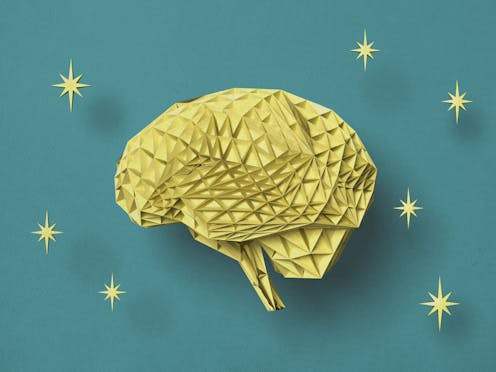
The human brain has been called the most complex object in the known universe[1]. And with good reason: It has around 86 billion neurons and several hundred thousand miles of axon fibers connecting them.
Unsurprisingly, the process of brain folding[2] that results in the brain’s characteristic bumps and grooves is also highly complex. Despite decades of speculation and research, the underlying mechanism behind this process remains poorly understood. As biomechanics[3] and computer science[4] researchers, we have spent several years studying the mechanics of brain folding and ways to visualize and map the brain, respectively.
Figuring out this complexity may help researchers better diagnose and treat developmental brain disorders such as lissencephaly, or smooth brain, and epilepsy. Because many neurological disorders[5] emerge at the early stages of development, understanding how brain folding works can provide useful insights into normal and pathological brain function.
The mechanics of brain folding
The brain is made of two layers[6]. The outer layer, called the cerebral cortex, is composed of folded gray matter made up of small blood vessels and the spherical cell bodies of billions of neurons. The inner layer is composed of white matter, consisting mostly of the neurons’ elongated tails, called myelinated axons.
In recent years, researchers have shown that mechanics[9], or the forces that objects exert on one another, play an important role in the growth and folding of the brain.
Among the several hypotheses that scientists have proposed to explain how brain folding works, differential tangential growth[10] is the most commonly accepted because it’s well-supported by experimental observations[11]. This theory assumes that the outer layer of the brain grows at a faster rate than the inner layer because of how neurons proliferate and migrate during development. This mismatch in growth rates puts increasing amounts of compressive forces on the outer layer, leading to overall instability of the growing brain structure. Folding these layers, however, releases this instability.
To better explain this theory, Jalil made a mechanical model[12] of the brain that assigned a greater growth rate to the outer layer than the inner layer. As expected, this mismatch in growth rates caused the inner layer to block the outer layer from spreading out. Because the outer layer can’t expand further because of this blockage, it has to fold and buckle inside the inner layer to reach a more stable structure.
Another study[13] using a 3D-printed hydrogel brain model also showed that a mismatch in growth rates results in folding.
Researchers from Harvard used a hydrogel model to show that folding reduces instability from differential growth rates of the inner and outer layers of the brain.This buckling happens because folding maximizes the brain’s surface-to-volume ratio, or the amount of surface area the brain has relative to its size. A higher surface-to-volume ratio allows the brain to pack in more neurons in a given space while decreasing the relative distance between them.
Jalil’s research team has also found that other mechanical factors[14] also affect the eventual shape a developing brain will take, including the brain’s initial outer layer thickness and how stiff the two layers are relative to each other.
More recently, our simulation studies have shown that axons, the part of the neuron that helps it transmit electrical signals, play a role in regulating the brain’s folding process[15]. Our model showed that brain ridges formed in areas with a high number of axons, while valleys formed in areas of low axon density. We confirmed these findings with neuroimaging and tissue samples from real human brains. This reinforces the importance that axon density plays in brain development and may speak to the origins of conditions like autism[16] and schizophrenia[17] that have irregular brain structure and connectivity.
Both of us are now in the process of developing more sophisticated models of the brain[18] based on neuroimaging of real brains that will provide an even more detailed simulation of brain development.
The mechanics of brain disorders
Our brain models provide a potential explanation for why brains may form abnormally during development, highlighting the important role that the brain’s structure plays in its proper functioning.
Brains with abnormal folding patterns can result in devastating conditions. For example, a brain model with a thicker than usual outer layer forms fewer and larger ridges and valleys than one with normal thickness. At the extreme, this can result in a condition called lissencephaly[19], or smooth brain, that has a complete absence of brain folds. Many children with this condition have severely stunted development and die before age 10.
On the other hand, polymicrogyria[20] has a thinner than usual outer layer and results in an excess of folding. This condition has also been replicated through mechanical modeling[21]. People with this condition can have mild to severe neurological problems, including seizures, paralysis and developmental delays.
Scientists have also identified abnormal folding patterns in brain disorders such as schizophrenia[22] and epilepsy[23].
Next steps in brain mechanics
Understanding the mechanisms behind brain folding and connectivity will provide researchers with the knowledge foundation to uncover their role in developmental brain disorders. In the long term, clarifying the connection between brain structure and function may lead to early diagnostic tools for brain diseases[24].
In the future, artificial intelligence[27] may be able to give even more insight about the normal growth and folding of the human brain. But even with all these advancements in neuroscience, researchers like us have our work cut out for us as we continue trying to decipher the mystery of the most complex known structure in the universe.
[Too busy to read another daily email? Get one of The Conversation’s curated weekly newsletters[28].]
References
- ^ most complex object in the known universe (www.npr.org)
- ^ brain folding (doi.org)
- ^ biomechanics (scholar.google.com)
- ^ computer science (scholar.google.com)
- ^ neurological disorders (doi.org)
- ^ two layers (www.spinalcord.com)
- ^ Emma Vought (doi.org)
- ^ CC BY (creativecommons.org)
- ^ mechanics (www.britannica.com)
- ^ differential tangential growth (www.jstor.org)
- ^ experimental observations (doi.org)
- ^ mechanical model (doi.org)
- ^ Another study (doi.org)
- ^ other mechanical factors (doi.org)
- ^ regulating the brain’s folding process (doi.org)
- ^ autism (doi.org)
- ^ schizophrenia (doi.org)
- ^ developing more sophisticated models of the brain (www.nsf.gov)
- ^ lissencephaly (www.ninds.nih.gov)
- ^ polymicrogyria (medlineplus.gov)
- ^ mechanical modeling (doi.org)
- ^ schizophrenia (doi.org)
- ^ epilepsy (doi.org)
- ^ early diagnostic tools for brain diseases (doi.org)
- ^ jgmarcelino/flickr (www.flickr.com)
- ^ CC BY (creativecommons.org)
- ^ artificial intelligence (www.nature.com)
- ^ Get one of The Conversation’s curated weekly newsletters (memberservices.theconversation.com)
Authors: Mir Jalil Razavi, Assistant Professor of Mechanical Engineering, Binghamton University, State University of New York